Articles on this
page by Collyn Rivers
CABLE SIZE &
CONVERSIONS
CABLE CONVERSION
TABLE
VOLTAGE DROP
LEAD ACID BATTERIES
WHAT SOLAR MODULES
REALLY PRODUCE
Cable
Sizes & Conversions
by
Collyn Rivers
All
electrical cable resists the flow of current
and the larger the
diameter of the cable, and the shorter its length, the lower is its
resistance. It is essential to minimise this resistance because it
reduces the voltage that lights, refrigerators and other electrical
bits and pieces need to work correctly.
In practice, the highest
acceptable voltage loss in any 12/24 volt campervan or motorhome
wiring is 3% of the battery voltage. That is: 0.36 volts, and 0.72
volts in 12 and 24 volt circuits respectively. Professional
installers often work toward 5% losses because it saves on cable
costs – but at the expense of their clients whose electric fridges
will never work as well as their makers intended.
Given that 3% voltage
drop is the maximum acceptable drop (but 1.5%-2% is better) it
should be easy enough to select cable accordingly: tables of
recommended cable sizes for various current flows are readily
available. There’s also a really simple bit of arithmetic that does
the job superbly (see below).
Auto
Cable – a Trap!
Unfortunately there’s a huge trap. Automobile cable is commonly sold
using a rating system that appears to correspond (but doesn’t) with
appliance manufacturers’ cabling recommendations. Nor does it, nor
indeed can it, correspond with other cable size standards.
Auto cable is that cable
invariably sold by auto parts stores, auto-electricians and many
hardware stores. This cable is rated in terms of its outside
diameter – insulation and all. In other words all that
measurement tells you is the size hole it can be passed through!
But most appliance
manufacturers and virtually all electrical trades specify cable
either directly in terms of the cross-sectional area (in sq.mm) of
its copper conductor - or by a numbering system (such as AWG) that
relates to conductor area.
A typically recommended
cable size is 2.5 mm.sq. Generally, the appliance maker will spell
this out in full (eg. 2.5 sq.mm) – but not invariably. You can
however be certain that if the appliance maker talks about 2.5 mm
cable they refer to 2.5 sq mm cross-sectional area.
But
when a buyer fronts up to the parts store and asks for the specified
2.50 mm cable what he will almost certainly be sold is not 2.50
mm.sq.
cable, but 3.00 mm diameter auto cable (the closest made to
2.5 sq mm). A 3.0 mm diameter auto cable’s conductor area
however is typically a tad over 1.0 sq mm – about one third of
the required size
If such cable is used for
example for fridge wiring, the voltage drop across it will be close
to three times that specified as the maximum by the appliance maker!
Unless that cable is
exceptionally short, that fridge, which may be a top brand in
perfect working order, will never work satisfactorily. This is the
main reason why so many 12-volt fridges disappoint, and why trailer
batteries are often grossly undercharged. Yet countless (in my
experience, most) trailers and motorhomes are, at least in
part, wired this way! And that includes professional
installations.
Ampacity
Compounding this, automobile cable may also be rated in amps. Thus,
‘3 mm’ cable is highly likely also to be described as ’20 amp’ (the
amount of this claim varies from maker to maker). Most people
not unreasonably take this as the recommended current rating. But
it’s not.
That ’20 amp’ rating is
not a recommendation of the current the cable could/should carry. It
is an indication of the highest current that cable can carry before
its insulation virtually melts (this rating is often known as ‘ampacity’).
It does not take voltage drop in to account. Ampacity is a
fire-related thing only.
How to
Choose the Right Cable
As
noted previously, appliance makers usually specify the minimum-sized
cable that may be used. Some supply the actual cable. In all cases,
heavier cable must be used if the length of cable is longer than
that specified.
But this is of no help to
someone wiring a complete vehicle. The rule here is to assess the
maximum current that will be carried by each cable run and specify
that cable size accordingly.
Tables of cable sizes for
various lengths of cable and current carrying capacity are
obtainable from cable manufacturers, are also published in books
such as my own ‘Motorhome Electrics’. I have included a simplified
version in this feature
There is a slight discrepancy in most published
conversions (mine are corrected) between the most commonly used AWG
gauges and ISO gauges. This discrepancy, due to ‘rounding up’, of
the two gauges has resulted in the general use of cables one AWG
gauge too small. To be safe, use one AWG gauge size larger (ie.
numerically lower than most conversion tables shows. Note the one
reproduced here is correct
ISO gauges are easy. The
actual gauge number (eg. 2.5, 4.0, 6.0, 10 etc) is the actual cross
sectional area, of the conductor, in mm.sq.
It is practicably
impossible to give any meaningful conversion between auto cable, AWG
or ISO. This is because the insulation thickness of auto cable
varies from vendor to vendor. Thus 4 mm auto cable can does have
conductor cross-sections from an extraordinary 1.25 sq mm – a more
typical 1.8 sq mm. A few makers supply it at 2.0 sq mm.
What can be assumed is
that 4 mm auto cable can be used instead of 1.5 sq mm cable; 6 mm
auto cable can be used instead of 4 sq mm cable, 8 mm auto cable is
almost the same as 8 sq mm. Anything smaller than 3 mm auto cable
has so little copper that it’s really only useful for instrument
wiring.
Just why automobile cable
is sold using this insane and misleading rating is unclear. The
sooner it changes the better as it has caused huge problems for
countless people for decades.
A
Better Way
As
mentioned previously is also very easy to work out the voltage drop
for any given length of cable, current flow, and cable size. I
always use this rather than published tables (including my own)
because once remembered it’s so easy to do – and it gives the right
answer every time. The formula is simply:
Voltage drop equals (cable length (in metres) X
current (in amps) X 0.017) divided by cable cross-section in mm.sq.
For example: 10 metres X 5 amps X 0.017 = 0.85.
Divided by (say) 2.5 (sq mm), the voltage drop is 0.34 volt. This is
just acceptable – but (here) 4.0 sq mm would be even better.
That results in a drop of 0.212 volts.
Cable size tables and the
above formula is for the voltage drop across a single
conductor path. Where, as is common with trailers, there’s twin
conductors (one positive, one negative) the total conductor
length must be taken into account. In other words, if there’s a five
metre run using twin cable, that’s 10 metres to be taken into
account.
The very best cable to
use for campervan and motorhome work is so-called ‘tinned’ copper.
It’s not actually tinned, but rather copper electroplated with a
nickel alloy. It is obtainable from boating electrical equipment
suppliers and wholesale electrical suppliers, but is hard to locate
otherwise. It’s worth tracking down, not least because it is
available in ISO sizes.
The above is extracted
from my previously published articles and books. The theme is
greatly expanded in my book ‘Motorhome Electrics – and Caravans
Too!’ and this book is being increasingly used as a reference work
by auto electricians
Australia-wide. It is
also now being used a the basic text in this field by TAFE.
It also sells in
large numbers overseas – especially in New Zealand and the UK.
Copyright
Please note that ALL my published writing
is fully protected under the Commonwealth Copyright Act. This
article and (copyright) Table is reproduced here by express
permission from the Copyright Holders – Caravan and Motorhome Books,
Broome, WA 6725. It may not be reproduced in any shape or form, or
in any media without express permission as above.
Caravan and Motorhome Books
There is a whole heap of
information on various subjects on my website:
Caravan
and Motorhome Books by Collyn Rivers
Collyn Rivers
Broome, August 2004.

Cable
Conversion Table
Sq.mm - ISO |
0.75 |
1.0 |
1.5 |
2.5 |
4.0 |
6.0 |
10 |
16 |
25 |
35 |
50 |
70 |
95 |
120 |
150 |
Auto
Cable |
2.5 |
3.0 |
4.0 |
5.0 |
6.0 |
|
8.0 |
|
|
|
|
|
|
|
5/0 |
AWG |
18 |
17 |
15 |
14 |
12 |
10 |
8 |
6 |
4 |
2 |
1 |
2/0 |
3/0 |
4/0 |
5/0 |
B&S |
18 |
17
|
15 |
14 |
12 |
10 |
8 |
6 |
3 |
2 |
0 |
2/0 |
3/0 |
4/0 |
|
Note: this table is copyright ‘Solar That Really Works' third
edition, 2012.
Caravan
and Motorhome Books by Collyn Rivers
back to top

Voltage Drop
by Collyn Rivers
Current |
<1 metres |
<2 metres |
<3metres |
<4 metres |
<5 metres |
<6 metres |
5 amps |
1.0 (16) |
1.0 (16) |
1.5 (14) |
2.5 (12) |
2.5 (12) |
4.0 (10) |
10 amps |
1.0 (16) |
2.5 (12) |
2.5 (12) |
4.0 (10) |
6.0 (8) |
6.0 (8) |
15 amps |
1.5 (14) |
4.0 (10) |
6.0 (8) |
6.0 (8) |
10 (6) |
10 (6) |
20 amps |
2.5 (12) |
4.0 (10) |
6.0 (8) |
10 (6) |
10 (6) |
16 (4) |
30 amps |
4.0 (10) |
6.0 (8) |
10 (6) |
16 (4) |
16 (4) |
25 (2) |
50 amps |
6.0 (8) |
10 (6) |
16 (4) |
25 (2) |
25 (2) |
25 (2) |
Cable sizes for 12-volt fridge and other
circuits. The sizes, in square millimetres, (and in brackets
AWG) introduce less than 3% voltage drop at the
maximum distance in each range. In the above Table, 4 mm auto
cable can replace 1.5 sq mm, and 6.0 mm auto cable can replace any
size up to 4.0 sq mm.
Caravan and Motorhome Books
Caravan and Motorhome
Books by Collyn Rivers
back to top

Lead
Acid Batteries
by Collyn Rivers
Batteries are like teen-agers. They come in different shapes and
sizes, some are prone to sulking, others behave like (flawed?)
angels, but by and large their behavior reflects how they are
treated.
Starter
batteries, and deep cycle batteries work in a basically similar way.
Energy is stored within them as a result of an electro-chemical
reaction between lead plates and a water/acid mix (called the
electrolyte).
Charging is effected by imposing a voltage across
the battery that is greater than the voltage ‘within’ that battery.
The greater that voltage difference the quicker and deeper the
battery will charge. When battery voltage reaches the charging
voltage, charging ceases.
A starter battery delivers a very heavy current
(up to 400 amps). It does this (hopefully) for only a few seconds.
This discharges it by a mere 2%-3% and that small amount of energy
is replaced within a minute or two of the engine starting. In
practice, starter batteries spend most of their life somewhere
between 65% and 70% of full charge. Lead/calcium batteries may be
charged a bit higher.
Providing such heavy current requires the lead
plates to present a large surface area to the electrolyte. To enable
this, starter batteries have a large number of thin plates, but
whilst this form of construction allows heavy currents to flow for a
few seconds, it will not withstand more than a few extended
discharges. Flatten most starter batteries half a dozen times and
they are dead
Deep Cycle Batteries
A deep cycle battery differs from a starter
battery in that it is intended to operate consistently over a much
large part of its capacity. It cannot however supply remotely as
heavy a current, in fact it will be damaged if discharged regularly
at greater than 25% or so of its amp/hour capacity.
Despite their name, deep cycle batteries cannot
consistently provide remotely the energy that the label on the side
may lead you to believe. Without adequate solar or ‘smart’ battery
charging a deep cycle battery is unlikely to exceed 70% of nominal
charge. So there’s 30% lost up front. Worse, battery makers advise
not to discharge below 50%. As that leaves only 20% for actual
use almost everyone ignores the battery makers’ advise and many
people routinely run them down until the lights go dim (about 80%
discharged).
If treated as above, the best deep cycle battery
yet made is good for 80-100 charge/discharge cycles. If run down to
about 30% remaining charge they are good for 150-200 cycles. Doing
as the makers advise gives you 500-1000 cycles.
Sadly, magazine article after another (and
advertisers who should know better) routinely claim a fridge that
(for example) draws 5 amps will routinely run for 20 hours on a 100
amp/hour battery. You’ll see pigs flying in formation (whilst
singing arias from Tosca) before a 100 amp/hour battery can do that!
The reality is that ‘deep cycle’ batteries would better be described
as ‘less-shallow cycle’ batteries.
How Low Should I Discharge?
Because the amount of air shifted by a cooling
fan is proportional to the cube of its rotation, low voltage doesn’t
do a great deal for fan-cooled motors, but otherwise running the
battery way down is unlikely to do any harm. Most fridges have a
voltage-sensing cutout that disconnects the incoming power if it
drops (typically) below 11.4 or so volts. This is usually promoted
as for protecting the battery, but its main job is to protect the
fridge motor from overheating.
Discharge level is essentially a trade-off
between convenience and your bank account, but unless you keep the
discharge to 65% (i.e. 35% left) you are better off buying the
cheaper traction batteries. These, and true deep cycle
batteries are likely to last about as long. But please do not take
the above as a recommendation to discharge batteries below 50%. I’m
simply explaining what happens if you do.
A far better way is to have a charging setup
(which will generally require solar) such that the batteries are
routinely charged close to 100% and discharged overnight by a
probable 15-20%. This way the batteries stay much of the time around
85%-90% fully charged – and last forever. That’s how my OKA works
and its last set of deep cycle batteries lasted over seven years.
(Just how to do this and why, is explained in my book ‘Solar That
Really Works!’ I have published it in separate ‘Caravan’ and
‘Motorhome’ editions.
Microwave
Ovens - A Trap
Be aware that an ‘800-watt’ microwave oven may
draw, via a 12-volt inverter, close to 150 amps. Such heavy current
damage will damage any deep cycle battery bank of less than 350-400
amp/hours.
Campervans and small motorhomes commonly have
microwave ovens, yet may have a battery of only 150 amp/hr. If you
use that microwave away from mains power, a marine battery is a
better bet. This is an exceptional recommendation however as a
marine battery’s capability, of delivering light starter current yet
retaining some deep cycle characteristic, is of no benefit in normal
use.
Knowing What’s Left
In a deep cycle battery, especially when cold,
the ‘charge’ held on the lead plates massively leads what’s
happening in the electrolyte. And vice versa. This is because the
electro-chemical reactions are very slow. Because of this, any
measurement of voltage taken within 12 hours of charging or
discharging is all but meaningless. All you are measuring is the
surface voltage of the plates.
Hence, a substantially ‘flat’ battery will
present as close to fully charged (>12.6 volts) for a long time
after being fast charged for minute or two. And an almost fully
charged battery may present as near enough to ‘flat’ (<11.8 volts)
after driving a microwave oven for a few minutes.
Despite this any camping site will have people
‘checking’ batteries during the day and making hugely incorrect
assumptions on the basis of what they find. Even doing this
first thing in the morning, with absolutely everything having been
turned off overnight, may still result in 30% or more error. Further
one needs a very good meter to have the accuracy and resolution to
measure the critical 0.2 –0.3 volt in 12.7 or so volts.
A hydrometer reading is better – but the battery
still needs to have rested. Take note
only of the hydrometer’s specific gravity reading, NOT of the
coloured bands and indications thereon. These markings usually
relate to starter batteries and indicate CHARGED at 1.280 SG or
more. A deep cycle battery is close to fully charged at an SG of
1.250 and is likely to be high as it will go by 1.260. Also, as
ambient temperatures rise, batteries charge at lower specific
gravities and voltages.
Measuring What’s Left
As a very rough guide, a well-rested deep-cycle
battery that shows 12.25 volts off-load is about 50% charged. Full
charge is likely to be 12.6-12.8 volts but the slightest load may
pull that down to 12.55 or lower. Table 1 gives a rough guide, but
only after batteries have rested for at least 12-hours.
The only really effective way of knowing
remaining charge is to measure what goes in and what comes out and
deduct charging losses. What’s left is more or less what you’ve got.
It’s actually a bit more complex than that as the rate of
charge/discharge affects the result. This was thoroughly explained,
via Peukert’s Equation, back in 1897 (email me if you’d like a
copy).
Almost every good solar regulator costing over
$300 or so shows what comes in and what goes out, but do not
necessarily include the Peukert correction (although the Mastervolt
unit does). Stand-alone energy monitors are also available but
if you are going to use solar it’s far cheaper to use the functions
provided by the more up-market solar regulators.
Sealed Batteries
Traditionally, the term ‘sealed battery’
described gel cells and, in recent years, AGM (Absorbed Glass Mat)
batteries. These batteries are heavier, bulkier and costlier
than conventional lead acid batteries. They charge close to 100% via
standard alternators and may be discharged deeper with far less
self-damage. They thus provide much closer to 100% of their nominal
capacity and this compensates substantially for their greater
weight, bulk and cost.
In recent times the term ‘sealed’ battery has
also become a synonym for ‘low maintenance battery’. These batteries
use a small proportion of calcium, and other things, in their plates
to reduce gassing and water consumption. That, plus a larger
reservoir above the plates, enables them to be permanently sealed.
This seems a good ideas for starter batteries (which have an
intended life of only two/three years) but I am not yet convinced of
their suitability in deep cycle form – where a well-maintained and
correctly used conventional battery can last five to seven years –
unless you prefer to swap battery longevity for freedom from
checking the electrolyte level every eight-twelve weeks.
Charging Batteries
A vehicle charging system is deliberately
designed to drastically cut back charging at 70% of full charge. The
only really safe ways to approach 100% charge (90% is a realistic
target) is via adequate capacity solar modules and solar regulator;
or via a ‘smart’ charger – also increasing known as a three-stage
charger.
These latter chargers are not cheap – they start
at $300 or so – but so far most of their vendors’ seem to have
overlooked that a 10-amp smart charger will charge a lead acid
battery as fast as, and deeper, than any chain-store 20-amp charger.
Smart chargers may be left permanently ‘on’. If this is done the
batteries will last many times longer. Thus, despite their cost,
smart chargers saves you money in the medium-long term.
Like most things in life , with batteries one
tends to get back less than you put in – about 15% of the
charge/discharge cycle is lost in heat. This needs to be remembered,
but not always is, when installing solar.
Maintenance
Keep the terminals clean externally (a tablespoon
of bicarbonate of soda in a bucket of water works like a charm).
Once a year disconnect the terminals, clean them until shiny on
their contacting surfaces. After reconnecting, coat with Vaseline or
battery protection goo.
Check water levels at least every eight–ten
weeks. A correctly charging battery should
use some water. About one centimetre every ten weeks is normal in
temperate climates. If less the batteries are probably being
undercharged. If much more, and unless you are in a very hot area,
they are possibly being overcharged.
Avoid Christmas trees of cables hung off battery
terminals. Instead, install one or more common power posts, and take
a single heavy cable from there to the battery terminal.
There’s a huge amount more one can write about
batteries (I know this because I already have in Motorhome
Electrics!) but the above gives a run down on the more important
aspects of their choice, care and feeding.
This article is published by
express permission of the copyright holder.
Please note that all of
Collyn Rivers’ published writing is protected under the Commonwealth
Copyright Act. No part may be produced in any form without the
written permission of the copyright holder (Caravan and Motorhome
Books, Broome WA).
See also my website
www.caravanandmotorhomebooks.com
Percentage Charge |
Voltage |
SG (<25 degrees) |
SG (>25 degrees) |
100% |
12.75 |
1.250 |
1.240 |
90% |
12.65 |
1.235 |
1.225 |
80% |
12.55 |
1.220 |
1.210 |
70% |
12.45 |
1.205 |
1.195 |
60% |
12.35 |
1.190 |
1.180 |
50% |
12.25 |
1.175 |
1.165 |
40% |
12.10 |
1.160 |
1.150 |
30% |
11.95 |
1.145 |
1.135 |
20% |
11.85 |
1.130 |
1.120 |
10% |
11.75 |
1.115 |
1.105 |
Zilch |
11.65 |
1.100 |
1.090 |
TABLE 1
Approximate voltages and
specific gravities of deep-cycle batteries (rested for at least 12
hours).
Copyright 2003 Caravan and
Motorhome Books, Broome WA.
Caravan and Motorhome Books
Caravan and Motorhome
Books by Collyn Rivers
back to top

WHAT SOLAR MODULES REALLY PRODUCE
Solar modules can and will
only produce the output claimed by their manufacturers if they are
used in specific circumstances. Typical trailer/motorhome usage is
not one of them.
Outputs vary from type to
type, but in typical installations most solar modules produce a bit
over 70% of their apparently claimed output. Many modules have a
small panel on their rear face that shows what they actually
produce. For an '80-watt' module this is usually about 58 watts.
Whilst this may seem
misleading it is legally defendable. (The explanation is included
later in this piece).
Two Main Types of Module
There are two main types of
solar module: for the purpose of the first part of this explanation
they may be seen as Uni-Solar and the rest. One type is not
inherently better than the other. They simply have different
characteristics that cause them to be more effective in some
conditions than in others.
Most solar modules produce
less power as they heat up - as much as 20% in seriously hot places
like the north of Australia, and they start losing this power from
only a few degrees above freezing. Uni-Solar uses a different
technology that enables the modules to produce slightly more power
as temperature rises.
Because the power output of
most solar modules falls as they get hot, from around 35 degrees C
(95 F) onward, a 64-watt Uni-Solar module produces much the same
output as an 80-watt most anything else. As they are about 15%
cheaper in most countries this can sometimes be a considerable
benefit
Uni-Solar modules are also less affected by partial shadowing. If
most panels are shadowed by an area even as small as a human hand,
they lose virtually all of their output. Uni-Solar modules lose only
that area shaded.
No solar module will work in
complete shade. I need to spell that out because after I stated
(elsewhere) that solar modules produce a small output under
high-intensity full-spectrum light, this rapidly turned into 'Collyn
says they even work under street lights'. They don't.
The above would appear to be
an overwhelming argument for Uni-Solar. This is not necessarily so.
Their heat advantage is only really worthwhile above 25 degrees C
(77 F) or so. And a big downside for many is that, because they are
less efficient, they are much larger (close to twice the area) of
most large later. That's why I use Solarex 80-watt modules on my OKA
truck, and 28 by 64-watt Uni-Solar modules on my 5 hectares at home.
In practice it is safe to assume that you will
get 58 watts from an 80-watt most-anything module, and about 55
watts from a 64-watt Uni-Solar module.
The amount of energy you will
capture each day can be readily worked out by taking the true output
of the modules (or about 72% of what it says on the marketing
brochure) and multiplying that by so-called the Peak Sun Hours
typical for where and when you are going. Maps showing this can
obtained from meteorological offices, but their's need translating,
and (for Australia) from all of my books. Peak Sun Hours are
explained below.
For example, a single
‘120-watt’ module is likely to produce about 92 watts in most
places. Broome has a typical six peak sun hours a day (in summer) so
the output there would be about 552 watt/hrs/day (or about 42.5
amp/hrs/day).
Ideally solar modules should
face the sun, but flat roof mounting is surprisingly effective.
Whilst modules can be carried loose, they are readily stolen. In my
experience it is not worth arranging for tilting or tracking systems
in latitudes less than about 27 degrees. Adding about 20% more
module capacity will make up for any loss.
Heavy cloud and rain cuts
output by 50% or more. The highest output is typically on bright
days with scattered low cloud. Then, sun shines down, is reflected
from earth and bounced down again from those clouds.
Because most modules are
heat-sensitive it pays to mount them so there's an air space
beneath. Less important with Uni-Solar but air space provides
useful heat insulation in the vehicle.
A solar regulator is essential
for all but systems where a very small solar module (<20 watts)
float charges a battery bank of at least 250 amp/hr. Even then I’d
use one myself.
Technical Stuff
Solar modules are tested using
'Standard Operating Conditions.'
The output of solar modules is
obtained by plotting curves of voltage and current and from these
using whatever combination of those two parameters gives the highest
'number'. In practice this tends to between 17.0 - 18 volts. Thus a
module that produces 4.7 amps at 17 volts is rated at 80 watts.
Power being P = IV, that
module will produce 56.4 watts at 12 volts - and 65.8 watts at 14
volts.
In other words module output
is a function of the voltage developed across the load.
The industry's 'Standard
Operating Conditions' (SOC) measures output at a cell (not ambient)
temperature of 25 degrees C at an irradiance of 1 kW sq.m. As this
equates to an ambient temperature close to 5 degrees C, the SOC may
better be regarded as 'Standard Test Conditions'.
Manufacturers do however also
quote a separate NOCT (Nominal Operating Condition Temperature).
This gives an indication of
the actual cell temperature at 20 degrees C ambient, but at 80% of
the irradiance of SOC operating conditions, a wind speed of 1 m.s,
and the back of the module enclosure open to atmosphere. Under these
conditions the NOCT is typically 47-49 degrees C. temperature - or
looking at it another way, the cell is likely to be 25-30 degrees C
hotter than ambient temperature.
Under NOCT a typical
80-watt module produces about 59 watts.
Mono- and poly-crystalline
modules lose output at a rate of approximately 0.4%-0.5% per degree
C above about 5 degrees C (voltage drops considerably, current rises
slightly). Thus at 30 degrees C ambient, output of modules using
that technology is likely to be 15% down (ie. over and above the
loss due to working at 12-14 volts).
Amorphous technology modules tend to be increase output very
slightly with rising temperature.
The current output of modules
is usually shown in the technical data. It may be shown as ISC
(short circuit current), or as operating current. The latter is the
figure to use. True output wattage is the operating current times
the operating voltage. It must then be corrected for temperature.
Standard Operating
Conditions |
|
Pmax (maximum power) |
81.4 W. |
Voc (voltage open
circuit) |
212 V. |
Isc (current short
circuit) |
5.25 A. |
VPmax (voltage max
power) |
16.60 V. |
Ipmax (current max
power) |
4.9 A. |
Normal Operating
Conditions |
|
Pmax (maximum power) |
59.0 W. |
Ipmax (current max
power) |
4.0 A. |
This
is actual data from the rear panel of an 80-watt module.
Whilst
it is rare for modules to produce their full rated output (Pmax) it
is possible to achieve it (or very close to it) when driving a load
(such as a water pump) that will operate at the voltage at which
peak output was tested. It can also be achieved by using multi-point
tracking systems that, in effect act as 'electrical torque
converters'. They 'swap volts for amps' (actually a dc/dc convertion).
So whilst people tend to be confused by the rating system, it's both
technically credible and legal.
The term Peak Sun Hours (PSH)
is not my invention! It's used extensively in the photo-voltaic
industry but does not seem widely known by engineers in other
disciplines. In effect it's the number of hours of midday sun on a
clear day equivalent to the irradiation for that day. One Peak Sun
Hour equals 1 kilowatt/hour/square metre.
Full
details of what can be run from solar, required module and battery
capacity, installation (probably rather more than you wanted to
know!) are in my books - and in brief on my website,
www.caravanandmotorhomebooks.com
This article
is copyright Caravan and Motorhome Books, Broome 2004.
Email:
collynr@bigpond.com
It is reproduced by express permission.
Caravan
and Motorhome Books
All
information has been reproduced with the kind permission of Collyn
Rivers

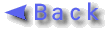
|